Modeling Electrotaxis of Stem Cells to Stroke Sites in the Human Brain
Research in vitro and in small animals has shown electric fields (EFs) induce directional migration in stem cells along an electrical potential gradient, a process known as cell electrotaxis, or cell galvanotaxis1,2. We built a first-pass COMSOL model to identify problems and demonstrate proof-of-concept of using two probes to direct endogenous stem cells to brain tissue damaged by stroke (Fig.1).
Figure 1. Two probes with electrode arrays to produce an electric field guiding stem cells to stroke sites.
Equations and Optimization Module
COMSOL’s AC-DC module Electric Currents interface solved these steady-state current conservation equations3,
∇ · J = 0 (1)
J = σE (2)
E = -∇ ∙ V (3)
where J is current density (A/m2), E
is electric field (V/m), V is voltage (in V), and σ is conductivity (S/m).
A minimum of 100mV/mm EF strength is required for efficacy (i.e. directed cell migration)1. Initial simulations used Global Inequality Constraints in the Optimization Module of 300 mV/mm as the optimal therapeutic EF strength1,2. Further, FDA-recommended safety limits for tissue stimulation include a maximum charge density of 30 μC/cm2 at electrode surfaces. Taken together, these values establish an initial ‘therapeutic window.’
Results and Discussion
DBS 3387 (Medtronic, Inc.) probe specifications used for modeling did not perform well for this application at 10mm probe separation intended for human patients, showing a rapid 1/r2 drop in EF strength (Fig.2), although current density stayed 80% below safety limits. However, at the distance of 2.8mm used in rodents, a therapeutic value of 200 mV/mm was reached, replicating empirical results, with EF values increasing to 1300 mV/mm near the probes (Fig.3).
We aim to establish an EF strength of 300 mV/mm at a probe separation of ~10mm while staying within FDA-recommended safety limits. Initial 2D modeling showed that increasing electrode surface area reduced the 1/r2 effect, allowing us to reach the optimal therapeutic EF strength (Fig.4). Fig.5 shows volume of tissue stimulated (VTS) in the therapeutic window between the two probes 10mm apart.
Conclusions
As neuromodulation and the electroceutical approach continue to grow, it has become increasingly apparent that novel, specialized electrode designs are often required for new applications. This project is in its early stages as we work to determine optimal probe and electrode composition and geometries that can allow for adherence to the therapeutic window using a two probes setup with a separation of ~10 mm.
References
1. Feng, J. F. et al., Electrical guidance of human stem cells in the rat brain, Stem Cell Reports, 9, 177-189 (2017).
2. Babona-Pilipos, R., et al., Biphasic monopolar electrical stimulation induces rapid and directed galvanotaxis in adult subependymal neural precursors, Stem Cell Res Ther, 6, 1-13 (2015)
3. Dokos S., Modelling Organs, Tissues, Cells and Devices Using MATLAB and COMSOL Multiphysics. Lecture Notes in Bioengineering. Berlin: Springer (2017).
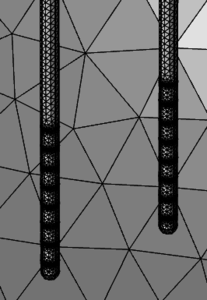
Download
- carlson_poster.pdf - 1.11MB
- carlson_electromed_presentation.pdf - 1.37MB